Paola Arlotta, Ph.D.
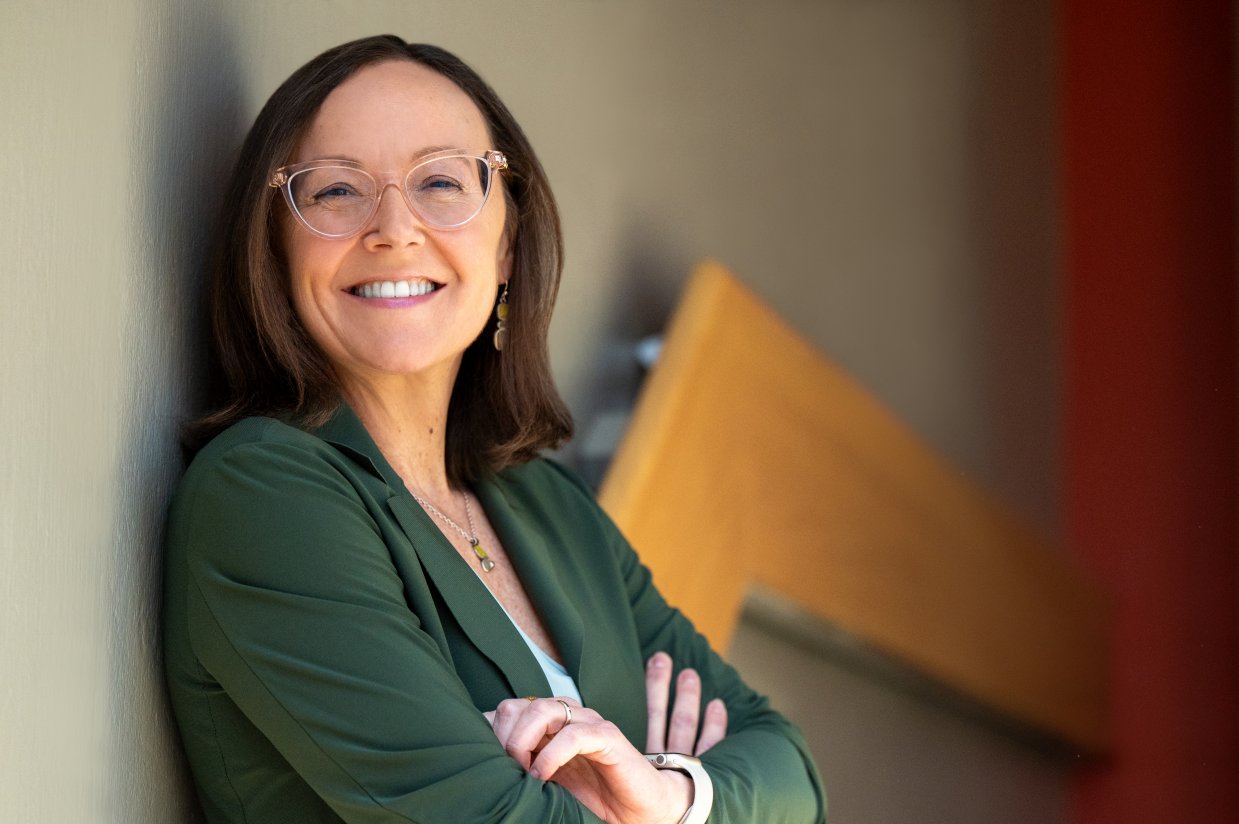
Dr. Paola Arlotta is the Golub Family Professor and Chair of the Department of Stem Cell and Regenerative Biology at Harvard University. She is a principal faculty member at the Harvard Stem Cell Institute, an institute member at the Broad Institute, and an associate member of the Stanley Center for Psychiatric Research at the Broad Institute.
The function of the brain relies on the integration into functional circuits of an outstanding diversity of cell types. Generation and maintenance of cell diversity, correct wiring into neural circuits, and orchestrated interaction of neurons and glia are critical, and when disrupted lead to neurological disease.
Focusing on the developing cerebral cortex, the Arlotta lab has had a long-standing interest in discovering the mechanistic principles that govern the establishment and maintenance of cellular diversity and its integration into working networks that subserve cortical function. While mice are instrumental for this basic work, the cortex has diverged dramatically in primates, and there is limited knowledge of development of the human cortex. Motivated by understanding how our own cortex develops and how human neurodevelopmental disease emerges, the lab has built on their basic program in the mouse to instruct, validate and study human cortical development in vitro, within 3D cortical organoids of unprecedented complexity and reproducibility.
Collectively, the Arlotta lab research program explores the interface between development and engineering of the neocortex, to gain fundamental understanding of both the principles that govern normal cortical development and of previously-inaccessible mechanisms of human neurodevelopmental disease.
Dr. Arlotta received her M.S. in biochemistry from the University of Trieste, Italy, and her Ph.D. in molecular biology from the University of Portsmouth in the UK. She subsequently completed her postdoctoral training in neuroscience at Harvard Medical School.
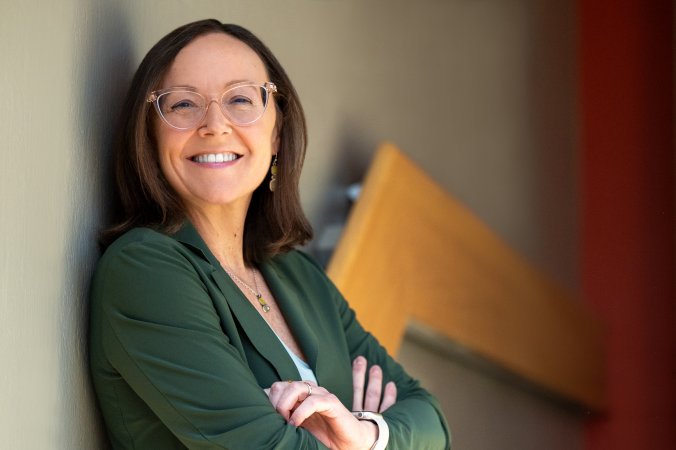
Paola Arlotta, Ph.D.
- Golub Family Professor of Stem Cell and Regenerative Biology
- Principal Faculty
Harvard Stem Cell Institute - Institute Member
Broad Institute of MIT and Harvard - Past Chair (2018-2024)
Harvard Department of Stem Cell and Regenerative Biology
Dr. Paola Arlotta is the Golub Family Professor and Chair of the Department of Stem Cell and Regenerative Biology at Harvard University. She is a principal faculty member at the Harvard Stem Cell Institute, an institute member at the Broad Institute, and an associate member of the Stanley Center for Psychiatric Research at the Broad Institute.
The function of the brain relies on the integration into functional circuits of an outstanding diversity of cell types. Generation and maintenance of cell diversity, correct wiring into neural circuits, and orchestrated interaction of neurons and glia are critical, and when disrupted lead to neurological disease.
Focusing on the developing cerebral cortex, the Arlotta lab has had a long-standing interest in discovering the mechanistic principles that govern the establishment and maintenance of cellular diversity and its integration into working networks that subserve cortical function. While mice are instrumental for this basic work, the cortex has diverged dramatically in primates, and there is limited knowledge of development of the human cortex. Motivated by understanding how our own cortex develops and how human neurodevelopmental disease emerges, the lab has built on their basic program in the mouse to instruct, validate and study human cortical development in vitro, within 3D cortical organoids of unprecedented complexity and reproducibility.
Collectively, the Arlotta lab research program explores the interface between development and engineering of the neocortex, to gain fundamental understanding of both the principles that govern normal cortical development and of previously-inaccessible mechanisms of human neurodevelopmental disease.
Dr. Arlotta received her M.S. in biochemistry from the University of Trieste, Italy, and her Ph.D. in molecular biology from the University of Portsmouth in the UK. She subsequently completed her postdoctoral training in neuroscience at Harvard Medical School.
Lab Overview
Most of our work aims at defining the molecular rules that shape and retain neuronal diversity in the cerebral cortex; understanding how pyramidal neuron diversity affects the behavior of other neurons and glia to build functional cortical circuits; and exploring the boundaries of the stability of postmitotic neuron identity in vivo.
While our work is rooted in development, we are also interested in understanding and modeling complex human cortical pathology, focusing on the development of new high-throughput in vitro models of human cortical development and neurodevelopmental disease using 3D cerebral organoids.