
Lee Rubin, Ph.D.
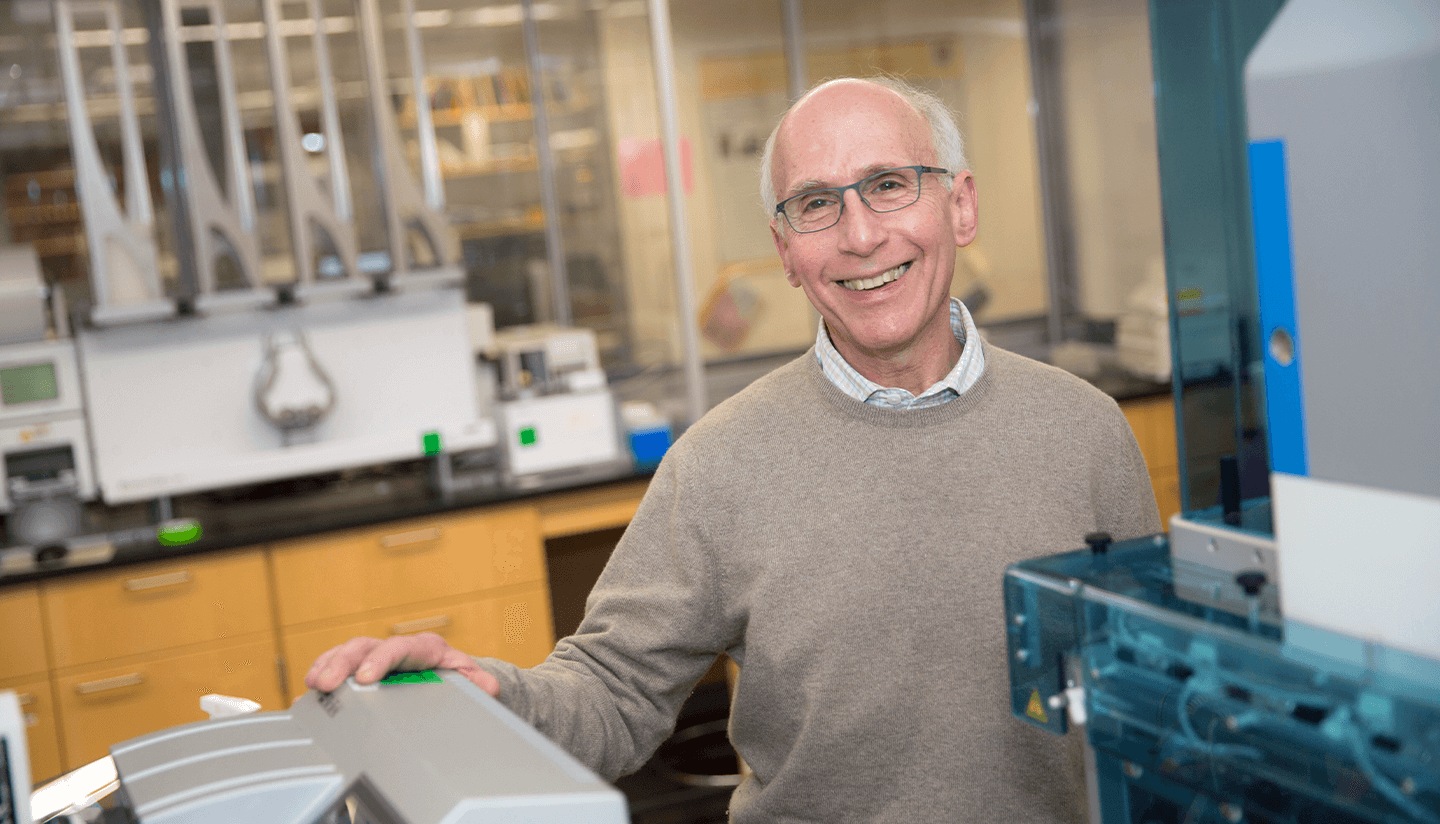
Our laboratory is broadly interested in the mechanisms underlying changes in the nervous system that occur because of aging or disease, as well as the interactions between the nervous system and the rest of the body. Studies involve a variety of in vivo, in vitro, and in silico approaches to generate insights into the complex molecular pathways that drive different disease states with a goal of identifying novel therapeutic insights. Current efforts in the lab include studies of the aging brain, CNS disorders (including neuromuscular and neurodegenerative) and skeletal muscle disorders.
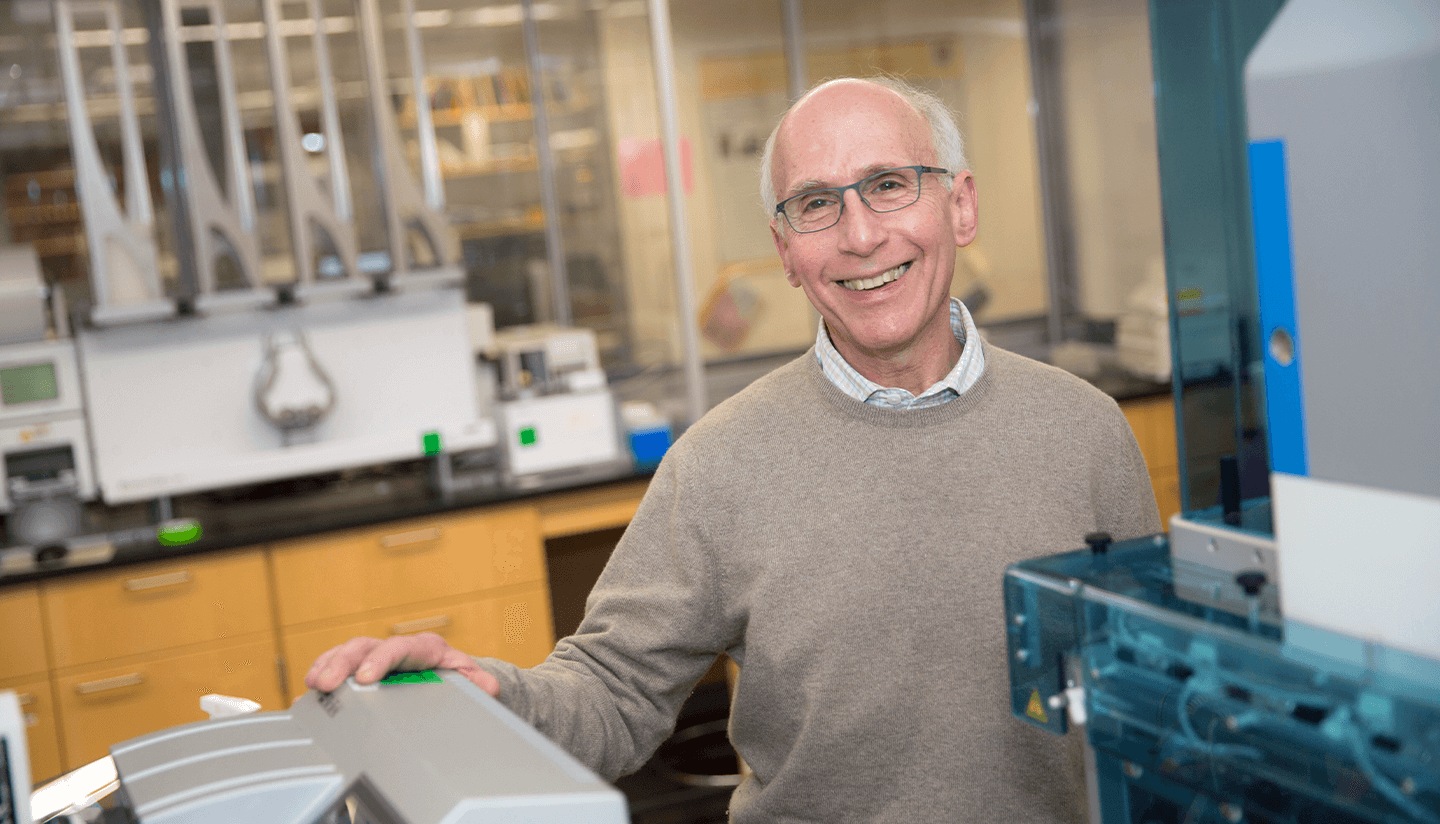
Lee Rubin, Ph.D.
- Professor of Stem Cell and Regenerative Biology
- Co-director, Harvard Stem Cell Institute Nervous System Disease Program
- Executive Committee Member
Harvard Stem Cell Institute
Lee Rubin is a Professor of Stem Cell and Regenerative Biology at Harvard University and Co-director of the Harvard Stem Cell Institute Nervous System Disease Program. He investigates the key molecular mediators of a variety of neurodegenerative diseases, with the ultimate goal of finding effective preclinical therapeutic candidates.
Dr. Rubin received his Ph.D. in Neuroscience from The Rockefeller University and completed postdoctoral fellowships in Pharmacology from Harvard Medical School and in Neurobiology from Stanford University School of Medicine. He has broad experience in both academia and industry, particularly in the realms of cell-based assays and drug discovery. Prior to coming to Harvard, Rubin was Chief Scientific Officer of Curis, Inc., a Cambridge-based biotechnology company, where his group identified the first small molecule regulators of the hedgehog signaling pathway. One of their antagonists was developed by Genentech and is now (as Erivedge) approved as the first oral treatment for metastatic basal cell carcinoma.
Lab Overview
The Rubin lab takes two main approaches. First, because neurological disorders are heterogeneous, lab members use patient iPSC-derived neurons to group patients into similarity clusters – sets of individuals who may respond better to particular therapeutics. To accomplish this, they employ large-scale production of neurons and non-neuronal cells, along with RNA sequencing, high content imaging and small molecule screening. A recent focus has been on formulating strategies to regenerate the neuromuscular system as a treatment for motor neuron and muscle disorders.
Second, in the last few years, the Rubin lab has also taken on a major challenge: namely, to find methods of reversing some of the effects of aging on brain function. Their work is driven by the observation that many physiological stimuli applied to peripheral tissues, such as the gut and muscle, can improve brain function by regulating the production of circulating blood factors. Lab members are combining multiomics, with AI/ML-driven computational analyses and behavioral measurements to identify single agents capable of producing meaningful improvements in patients with cognitive loss associated with aging or disease, For example, his research group discovered that a circulating protein, GDF11, has the ability to reverse some of the changes in the CNS associated with aging. They are actively exploring the therapeutic implications of these observations.
-
Aging of the Brain
Aging is the biggest risk factor for developing late-onset neurodegenerative diseases, such as Alzheimer’s disease (AD) and Parkinson’s disease. We are interested in identifying and studying the common biochemical changes that underlie aging and neurodegeneration.More about this work -
Skeletal Muscle
Neuromuscular disorders, such as Spinal Muscular Atrophy (SMA), often involve direct skeletal muscle defects in addition to the more well-studied spinal cord neuron degeneration. For example, SMA patients present with widespread muscle atrophy and weakness. Skeletal muscle tissue is known to regenerate rather well, a capability that is attributed to the activity of satellite cells, the resident skeletal muscle stem cell. We are carrying out studies to evaluate if defects in satellite cells contribute to neuromuscular disease. Our research combines the use of patient-derived iPSCs, genetic mouse models, and phenotypic drug screening methods.More about this work -
Small Molecule Screening Assays
We have established an extensive range of complex, image-based assays to probe the properties of stem cells and cells derived from them. We use automated, high-content screening imagers, associated robotic equipment, and selected small molecule libraries and combine this high throughput approach with detailed molecular studies to further our understanding of the mechanisms of disease and determine which compounds are most likely to be of therapeutic value.