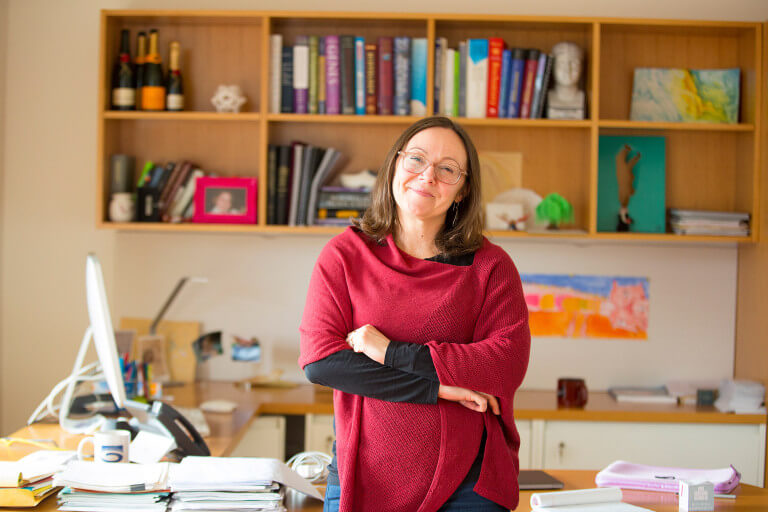
Human brain disorders have always presented researchers with a daunting challenge. They’re hard to study in laboratory mice because they affect the very organ that separates us from animals. And they’re difficult to study in humans because patient safety depends on noninvasive techniques.
Enter the brain organoid. Advances in stem cell biology and a new appreciation of the self-organizing powers of developing brain tissue have allowed researchers to create 3-D clusters of living brain that open a new window onto brain development and disease.
“I think that these brain organoids hold incredible potential for modeling human neurological disease in completely new ways,” said Paola Arlotta, the Golub Family Professor of Stem Cell and Regenerative Biology and chair of Harvard’s Department of Stem Cell and Regenerative Biology. “I like to imagine a future scenario where we will be able to ask very precise questions about what goes wrong in the context of psychiatric illness, for example.”
Arlotta has devoted her career to understanding brain development and what goes wrong in disease. She twice has stood accepted wisdom on its head. In 2013, challenging the theory that neurons cannot change, she used lab mice to show that one type of neuron can be transformed into another. A year later, she demonstrated that the insulating sheath of nerve cells, thought to be distributed identically along the axons of all neurons, instead displays distinct patterns in different cells. That led to the reinterpretation of some theories regarding the role of that insulation, called myelin, and how neurons use it in complex behaviors.
In recent years, Arlotta has become a pioneer in brain organoids, which she believes may one day shed light on little-understood conditions such as autism, schizophrenia, and bipolar disorder.
Arlotta collaborator Jeff Lichtman, the Jeremy R. Knowles Professor of Molecular and Cellular Biology, has held a front row seat to all this mystery. Through his “Connectome” project, he is working to build a map of neural connections by taking high-resolution images of thin brain slices.
“If getting to a full understanding of the brain is a mile, we have walked at least six inches,” Lichtman said. “You look at the actual structure of the brain or even an organoid and it’s just extraordinarily complicated. It’s much more complicated than anything humans have ever built. This is a little humbling.”
One consequence of that lack of understanding, Arlotta said, is that theories can stagnate.
“It is rather daunting,” Arlotta said. “How are we going to develop new treatments if we do not know what cell types, among the thousands present in the brain, are involved in psychiatric illness? How are we going to find molecular targets for new drugs if we cannot study the very organ that is affected? This is particularly problematic when diseases that start in the womb, during brain formation, manifest later in life.”
Arlotta said that creating organoids from people afflicted with brain disorders is akin to going back in time to watch how development plays out.
“What if we could somehow go back, so to speak?” she said. “What if we could take a sample of blood from a child with autism, make his or her own stem cells and turn those into a model of their brain? Could we then begin to watch in some small part how the brain had formed? And in so doing, will we have the unprecedented opportunity to shed light on what abnormalities have occurred?
“I think that if, in the next decade, we will have built on the use of brain organoids to understand what the neurobiological substrate of psychiatric illness may be, then we would look very proudly at the work that we are doing today.”
‘Liberating’ progress
Arlotta got her first glimpse of the potential for brain tissue to self-organize into 3-D organoids in 2012. She was watching a time-lapse video of human stem cells forming an early developing part of the eye called the optic cup. The video, recorded in the lab of Japanese scientist Yoshiki Sasai, showed development that seemed spontaneous, as if preprogrammed.
“We all stopped for a moment and watched — mesmerized — the video of a community of stem cells folding, changing, and self-assembling until a primitive retina was made,” Arlotta recalled. “That told us that the nervous system may have an incredible capacity for self-making. And that, to me, was the eye-opener. If stem cells can do that — make an optic cup, layer a human retina — perhaps stem cells know more than we think they do and, with minimal input from outside, perhaps they can form more complex regions of the nervous system, like some parts of the brain.”
When her lab began growing organoids from pluripotent stem cells about four years ago, she and her fellow investigators knew very little. The researchers tapped the expertise of Xander University Professor Doug Melton, who had begun growing pancreatic tissue, and borrowed protocols from other labs developing brain organoids to study early growth, a process in which pluripotent stem cells become brain tissue over the course of a few months.
Interested in later stages of development, Arlotta needed to figure out how to extend that growth, a task she handed to postdoctoral fellow Giorgia Quadrato.
Quadrato determined that a key variable is how many cells are initially used to “seed” the culture. She also tweaked the timing of when specific signaling molecules would be added to tell the stem cells to become brain tissue. By spring of this year, she had more than tripled the organoids’ longevity, allowing cells time to grow and differentiate.
“These organoids have self-organizing abilities; they know what types of cells to become,” said Quadrato, who recently joined the faculty of the University of Southern California. “The cells have built in themselves the program. They know how to differentiate, they know when to differentiate and what to become.”
To determine whether the cells her lab was growing related to those of the human brain, Arlotta turned to Lichtman, who for many years had been able to look at the organ in super-high resolution.
The goal was to identify protrusions on nerve cells called dendritic spines, where the synapses form that connect one cell to another. Embryonic nerve cells don’t have the spines, Arlotta said, so finding them would indicate the organoids were developing more mature neurons. It would also mean that the researchers could hope to explore a theory on schizophrenia that describes abnormal pruning of the spines in the teen years.
“The question was, do organoids have neurons, number one, and, number two, if they have neurons, do they have these features that are necessary to make the kinds of neural circuits found in normal brains?” Lichtman said. “Sure enough, there were dendrites and they had spines and the spines were studded with synapses of axons that were contacting them.
“So this was good news. It said these organoids to some degree were doing things that normal brains do.”
Arlotta’s lab has started to explore how the brain changes in autism, using CRISPR/Cas9 gene-editing techniques to insert genetic mutations associated with autism into stem cells and allowing the cells to develop into organoids to study side by side with those without that mutation.
“We have stem cells derived from patients with these diseases and already beginning to demonstrate that you can use organoids for the first time to tell what cell types are abnormal among the many, many in the brain,” Arlotta said. “That’s liberating.”
Organoids provide a glimpse into previously inaccessible aspects of brain formation, but they are very primitive and plagued by high variability, Arlotta stresses. Next for researchers will be learning how to better control their growth to produce reliable models for specific parts of the brain and the effects of specific conditions.
Alert to ethics
Though Arlotta strongly disputes characterizations of organoids as “mini-brains” or “brains in a dish” — descriptions that give the rice-grain-size lumps of tissue far too much credit, in her view — she acknowledges the ethical dimension of experimenting with the organ at the center of human consciousness.
It’s important to make sure that the research goes hand in hand with conversations that bring together scientists, ethicists, and society at large, she said. It’s also crucial, she said, that the science be communicated correctly, to prevent ethical concerns fueled by perception rather than data.
“There’s a need to be more rooted and real about what these models are,” Arlotta said. “These are not, I repeat not, ‘mini brains in a dish,’ and I think that nomenclature has negatively impacted the field. . . . It is important as a community to think about the implications of the work, but at the moment the risk is low.”
Ethical concerns also exist on the other side of the issue, she said, citing a responsibility to understand and treat psychiatric and neurodegenerative disease.
“We have the opportunity to study, understand, and inform treatment on human pathologies that have not seen a real new medicine in over 60 years, at a risk that is frankly not dissimilar to that of experiments that are very accepted in science, like the growing of human cells and neurons,” Arlotta said. “Should we not then take this opportunity to transform treatment?
“As a society, we must consider the ethical structure of this work. As a scientist, I must consider also the responsibility I have to make a difference, now that we can, for patients affected with some of the most devastating diseases of our time. Is it ethical or unethical not to take this opportunity to understand disease to alleviate suffering? I think the choice there is very clear.”