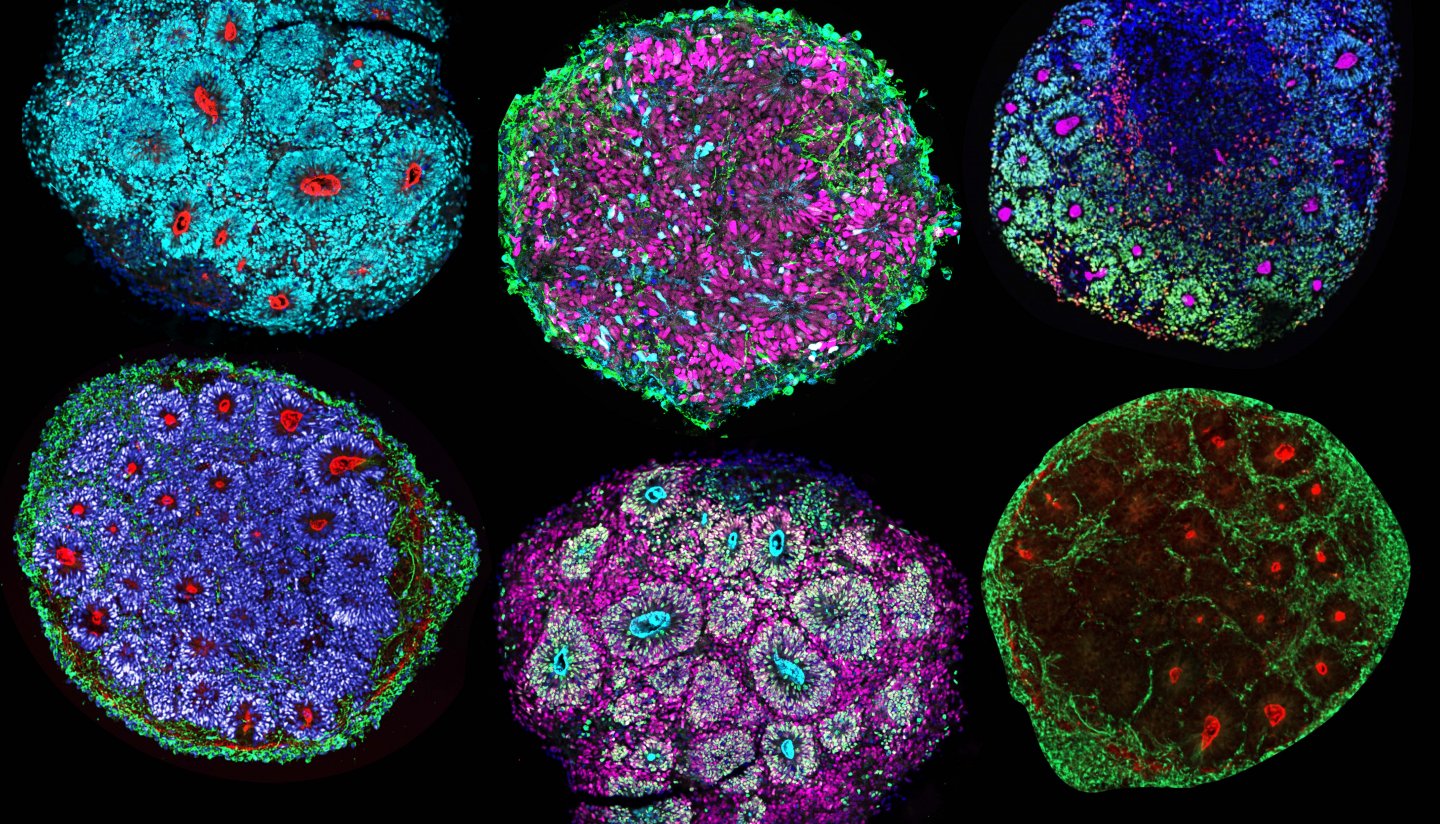
In a groundbreaking study led by Paola Arlotta, Golub Family Professor of Stem Cell and Regenerative Biology, researchers have developed a revolutionary platform that could change the way scientists study the human brain and its responses to various stimuli. This novel platform, relying on what the team calls ‘brain chimeroids’, offers a unique opportunity to understand how the cells of different human brains react to neurotoxic triggers, genetic mutations, and potential therapeutic compounds. The study, including significant contributions from colleagues at the Broad Institute, is published in Nature.
Chimeroids are advanced brain organoids composed of multiple types of cells of the brain. In this case, the team created chimeroids containing cells from multiple human donors, with the long-term goal of testing whether differences in responses by distinct people, including those with a family history of neuropsychiatric disorders, could be detected and studied using in vitro models of our brain.
“The idea of generating organoids that contain cells from multiple donors was directly motivated by our long-term interest in modeling psychiatric diseases, such as schizophrenia, and developmental disorders, such as autism spectrum disorder (ASD),” Arlotta explains. Most patients with these pathologies present risk linked to complex polygenic states rather than single gene risk. The team knew that leveraging this genetic information to understand the functional effect on the brain requires human models that can incorporate large numbers of individuals across a spectrum of polygenic risk.
“The ability to grow cells of distinct donors within the same organoid opens the door to exciting opportunities to investigate at scale and in a more controlled way the responses of the cells of the brain of many different individuals to many different types of stimuli, from genetic mutations, to infections, to drugs, the possibilities are endless,” Arlotta adds. This breakthrough means that instead of studying the effects of a compound on a single genetic background, researchers can observe how different genetic backgrounds respond to the same stimulus within the same organoid, paving the way for a multitude of future research possibilities.
The development of brain chimeroids builds on a decade of research by the Arlotta lab, which has pioneered the use of human in vitro systems, or organoids, to study neuropsychiatric disorders. “In this context, the goal was to develop a way of looking at different cells of the brain that are affected by a trigger in a more scalable way and develop a platform to read solid responses in these different cells,” adds co-first author Irene Faravelli, postdoctoral researcher in the Arlotta lab.
The process of creating the brain chimeroid involved several steps. First, pluripotent stem cells, which can be derived from any donor (healthy or patient), are differentiated in individual 3D cultures. In this case, cells from five individuals were included. After a period of time, the cells are coaxed towards neural progenitors, particularly those of a brain cortical fate. Next, the cells are removed from the culture, disassociated from each other, and reassembled into a 3D brain chimeroid that over time develops a multitude of cell types found in the human brain.
This approach ensures that the resulting brain chimeroids are composed of multiple brain cell types from multiple donors, each contributing to the overall structure. The researchers used cells from five different donors for this study, but the platform is designed to be flexible and scalable, potentially accommodating hundreds of donors in future studies.
This study exposed brain chimeroids to two well-known neurological compounds: ethanol and valproic acid. Ethanol exposure during pregnancy can lead to fetal alcohol spectrum disorder, while valproic acid, a drug used to treat mood disorders and seizures, has been linked to an increased risk of ASD when exposure occurs in the womb. The goal was to understand how different cells within the brain chimeroids responded to these compounds and whether there were variations in susceptibility among the different donors. Such interindividual differences are observed in the clinic, where exposure to these compounds does not have the same effect on every baby.
One of the most significant findings of this study is the ability to observe how specific cell types within the brain chimeroids respond to neurotoxic triggers. For instance, certain neurons or neural progenitors were more affected by ethanol or valproic acid exposure than others.
The readout is achieved through molecular transcriptomic analysis, which allowed the researchers to identify specific molecular pathways and cellular responses to the compounds. Such high-resolution data is crucial for understanding the variability in human brain responses and could have significant implications for personalized medicine.
The development of brain chimeroids was not without its challenges. One significant hurdle was ensuring that the cells from different donors maintained a balanced proportion over time. “A major technical challenge was that single donors would take over the culture over time, even if mixes were donor-balanced initially,” recalls co-first author Noelia Antón-Bolaños, postdoctoral fellow in the Arlotta lab. “Overcoming this challenge required a deep understanding of embryonic brain development and innovative computational analysis techniques.”
The team believes chimeroids have the potential to enable the study of complex polygenic states associated with psychiatric disorders like schizophrenia and ASD, or to predict responses to new medicine. Chimeroids could allow scientists to investigate brain cells of many patients, at scale. In addition, “Success in this area would mean that organoids could become pre-clinical trial ‘avatars’ informing on the differential responses of the cells of the brain of each individual to begin to predict clinical outcomes,” Arlotta explains. This could lead to more accurate preclinical trials and better predictions of clinical outcomes.
As Arlotta imagines, “What if one day we could use chimeroids as avatars to predict individual responses to new therapeutics before testing it in a trial? Or to stratify and better classify and diagnose patients to tailor more effective therapeutics to them? I like to imagine that future, frankly.”